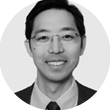
Corneal opacification is a leading cause of blindness and visual impairment worldwide.1 Causes of corneal blindness include degeneration, dystrophy, infection, inflammation, and trauma.2 When conservative measures prove inadequate, corneal transplantation is often the last line of defense. Globally, corneas are the most common and, owing to immune and lymphogenic privilege, the most successfully transplanted tissue.3
This article highlights several key areas of development that could disrupt the field of corneal transplantation.
THE EVOLUTION OF CORNEAL TRANSPLANTATION
Penetrating keratoplasty. The first human corneal transplant was performed by Austrian ophthalmologist Eduard Zirm in 1905.4 His rudimentary penetrating keratoplasty (PKP) countered the prevailing view of the day that selective replacement of corneal layers had a better chance of success than full-thickness corneal transplantation.4 PKP remains the dominant corneal transplantation technique worldwide,5 but there is a growing trend back toward lamellar techniques.
Lamellar techniques. Endothelial keratoplasty (EK) has overtaken PKP to become the most common keratoplasty procedure in the United States, with similar trends observed in other developed countries.2 The treatment for endothelial dysfunction has evolved from PKP to deep lamellar EK to Descemet stripping automated EK to Descemet membrane EK (DMEK). Only Descemet membrane and endothelial cells are transplanted in DMEK.
Surgical innovation has increased the accuracy of the anatomic replacement of diseased tissue. Both patients and surgeons have come to expect excellent outcomes, rapid visual rehabilitation and low rates of rejection and complications.6
Deep anterior lamellar keratoplasty (DALK) replaces the anterior 90% of the cornea while preserving healthy Descemet membrane and endothelium. The procedure has several advantages over PKP, including closed-globe surgery, increased tectonic integrity, and a reduced risk of allograft rejection.7 The adoption of DALK has been sluggish, however, plateauing the past decade. Barriers to adoption include misconceptions about the rarity of complications with PKP, the lack of a standardized DALK technique, and limited substantial benefits in terms of visual and refractive outcomes with DALK.7
Of the various DALK approaches developed to date, the big-bubble technique first described by Anwar and Teichmann is the most popular.6 Partial-thickness trephination is followed by the posteriorly angled insertion of a needle toward the central cornea and a forceful intrastromal injection of air to cleave the deep stroma from the pre-Descemet layer. DALK’s inherent challenges can be mitigated with technology. Intraoperative anterior segment OCT facilitates the accurate assessment of trephination depth and placement of the air cannula. Femtosecond lasers may be used to create precise trephination patterns and customized intrastromal tunnels for air injection.
A SHORTAGE OF DONOR TISSUE
The remarkable evolution in the field of corneal transplantation has occurred against a sobering backdrop: a tremendous global shortage of donor corneal tissue. Estimates suggest that up to 98% of patients who need a corneal transplant will not have access to this sight-saving treatment. Approximately 12.7 million people currently await a transplant, with 7 million in India and 2 million in China alone.5 According to the same report, just more than 180,000 corneal transplants were performed worldwide in 1 year—a fraction of the number of procedures required to meet demand. The COVID-19 pandemic brought the fragility of corneal transplantation—a donor-dependent solution—into sharper focus.3
The need for game-changing solutions is great.
REGENERATIVE MEDICINE AND CELL THERAPY FOR CORNEAL DECOMPENSATION
Fuchs endothelial corneal dystrophy (FECD) and bullous keratopathy are the leading causes of corneal decompensation, resulting in corneal edema, opacification, and eventually permanent scarring.2 Modern EK techniques can achieve excellent outcomes in these eyes. Current limitations, however, include a donor tissue shortage (as discussed earlier), a risk of rejection, a requirement for long-term topical immunosuppression, and the attendant risk of steroid-induced glaucoma. Two emerging approaches are regenerative medicine and cell therapy.
Regenerative medicine. Descemet stripping only is a regenerative medicine approach to patients with FECD in which the central 4 mm of Descemet membrane is carefully peeled off. The adjuvant use of a Rho kinase inhibitor has been shown to decrease the time to corneal clearance. Success rates have been as high as 85% to 90%.8
Another promising compound is TTHX1114 (Trefoil Therapeutics), a proprietary engineered fibroblast growth factor 1 variant. Fibroblast growth factor 1 stimulates cell proliferation and migration and protects against cellular stress and injury. TTHX1114 was used as an adjunct to Descemet stripping only in the phase 2 STORM study. Data presented at the World Cornea Congress 2022 showed a good level of safety and patients’ recovery of 20/40 visual acuity in a mean of 4.2 weeks with a single intracameral injection of the drug.9
Cell therapy. In 2018, Kinoshita et al published a landmark study in which 11 patients with FECD and bullous keratopathy received cell injection therapy of cultured corneal endothelial cells (CECs).10 The research group subsequently published encouraging 5-year safety and efficacy data.11 During treatment, donor cadaveric CECs were isolated and cultured in vitro. Together with a Rho kinase inhibitor, the CECs were injected into the anterior chamber of the recipient after the host endothelial cells had been gently debrided. The patient was then positioned face down for 3 hours.
In the human eye, CECs are arrested in the G1 phase of the cell cycle and do not proliferate in vivo.12 Under the right conditions, however, CECs can proliferate in vitro. Laboratory cell culture techniques could yield enough CECs from a single donor cornea for multiple treatments.12
An alternative to cell injection that also uses cultured CECs is to seed them onto a tissue-engineered graft and transplant it in a procedure similar to DMEK.13 Further clinical trials are required to assess the efficacy of this approach.
CEC culture and injection protocols require Good Manufacturing Practice–compliant facilities and highly trained scientists.
EK will likely remain the gold standard for treating corneal endothelial dysfunction for some time, but regenerative medicine and cell therapy approaches could become viable and less invasive options. If initial results prove replicable in subsequent trials, these treatment modalities could revolutionize the field and greatly alleviate the shortage of corneal donor tissue.
EXTRACELLULAR VESICLES FOR THE EARLY TREATMENT OF CORNEAL SCARRING
Visually significant corneal scars can arise from infection, trauma, and chemical injury. Approaches to mitigating the impact of these conditions under investigation include gene therapy delivering antifibrotic genes, microRNA therapy, and stem cell therapy.14 Mesenchymal stem cells (MSCs) in particular have an immunomodulatory ability. In animal models, MSCs have demonstrated an ability to prevent fibrosis and reduce the density of corneal stromal scars.14 Perhaps more significantly, the extracellular vesicles (EVs) of MSCs can affect target tissues in ways similar to their parental stem cells.
EVs are lipid bilayer membrane-bound vesicles excreted from cells and are vehicles for cell-to-cell communication. Common EVs include, in increasing size, exosomes (40–200 nm), microvesicles (50–1,000 nm), and apoptotic bodies (500–2,000 nm).15 MSC-derived EVs have antifibrotic, antiinflammatory, and even antiangiogenic properties. They could be delivered either in a topical eye drop or as an intrastromal injection. If proven effective, EVs would offer several significant advantages over corneal transplants and live stem cell therapy such as reduced complications, lower costs, and fewer regulatory challenges. Less stringent storage conditions, moreover, are required for EVs, and they could be administered in an outpatient setting.
The availability of an off-the-shelf product that can effectively treat visually significant corneal scars could significantly decrease the number of patients awaiting a corneal transplant.
CORNEAL ALLOGENIC INTRASTROMAL RING SEGMENTS AND KERANATURAL
Visual rehabilitation in keratoconus is approached in a stepwise manner. Options include spectacles or contact lenses, advanced surface ablation combined with CXL, phakic IOL implantation, and intrastromal corneal ring segments (ICRSs). When these modalities are insufficient, DALK or PKP is required. Keratoconus patients are often young, and corneal transplantation carries the risks of rejection, long-term failure, weakened corneal wound from trauma, and ocular surface disease.
Traditional ICRSs are made of PMMA and implanted in the midperipheral cornea to induce flattening and regularize corneal curvature. This produces a decrease in astigmatism and an improvement in patients’ visual acuity. Known complications of the technique are melt of the overlying cornea and extrusion. As described by Soosan Jacob, MS, FRCS, DNB, corneal allogenic intrastromal ring segments (CAIRS) are a modification of the technique that uses allogenic human donor corneal tissue.16 The tissue is deepithelialized, deendothelialized, and cut into the desired arc shape with a double-bladed trephine. The donor tissue is then implanted in channels in the midperipheral cornea cut with a femtosecond laser. Because of the reduced risk of device extrusion, the channels may be shallower than for ICRSs and thus have a greater capacity to induce flattening. An all-laser version of CAIRS has been reported in which the donor rings are fashioned with a femtosecond laser.17 CAIRS has been shown to induce regularization of the cornea, centralize the cone, and reduce regular and irregular astigmatism.
An issue with CAIRS is that it requires the use of transplant-grade corneal donor tissue, thus adding to demand. KeraNatural products (Lions VisionGift) are acellular rings and arcs derived from human donor corneas that are deemed to be unsuitable for transplantation owing to low endothelial cell counts. The rings and arcs are e-beam irradiated and sterile and have a shelf-life of 2 years.18 KeraNatural products have been used in CAIRS, reducing demand for donor tissue.
BIOENGINEERED GRAFTS
The development of synthetic, biologically compatible devices for restoring corneal clarity could significantly reduce reliance on human tissue.
EndoArt. A 50-µm thick, acellular, artificial endothelial prosthesis, EndoArt (EyeYon Medical), is made of a hydrophilic acrylic material. Clinical trials are ongoing. Early results indicated successful corneal deturgescence in eyes with poor visual potential (click here to read a related article).19 The off-the-shelf device operates on a simple barrier principle: It blocks the influx of aqueous from the anterior chamber. Compared to cadaveric donor corneal tissue, EndoArt can be stored for a much longer period and easily manipulated in the eye without concern about endothelial cell loss, and it does not require long-term topical immunosuppression. There have been reports, however, of partial or total detachment requiring rebubbling or fixation sutures.19,20
CorNeat Kpro. This synthetic cornea (CorNeat Vision) incorporates a PMMA optical member and an external biointegrating skirt formed by electrospinning carbonated polyurethane fibers. Unlike other keratoprostheses, CorNeat Kpro is designed to integrate with the conjunctiva rather than the avascular diseased cornea.21
Bioengineered porcine construct, double crosslinked. Rafat et al reported the results of a clinical trial in which bioengineered porcine construct, double crosslinked (BPCDX) was implanted in 20 patients with keratoconus during a femtosecond laser–enabled intrastromal keratoplasty procedure.22 The BPCDX was manufactured under Good Manufacturing Practice–compliant conditions. BPCDX has demonstrated excellent optical, biomechanical, biocompatible, and safety characteristics in laboratory and animal studies. Safety and efficacy were shown with 24-month postoperative follow-up. Mean reductions in maximum keratometry of 11.20 and 13.90 D were reported in the Iranian and Indian cohorts, respectively. Nineteen patients experienced an improvement in BSCVA or best contact lens–corrected visual acuity. An 8-week postoperative regimen of topical steroids was implemented, and no patient experienced inflammation, rejection, vascularization, or scarring.
CONCLUSION
Corneal transplantation has come a long way from the early 1900s. This article is by no means an exhaustive review of new concepts in the treatment of corneal blindness. The innovations discussed, however, represent exciting and potentially transformative developments in the field.
1. Robaei D, Watson S. Corneal blindness: a global problem. Clin Exp Ophthalmol. 2014;42(3):213-214.
2. Tan DTH, Dart JKG, Holland EJ, Kinoshita S. Corneal transplantation. Lancet. 2012;379(9827):1749-1761.
3. Ang M, Moriyama A, Colby K, et al. Corneal transplantation in the aftermath of the COVID-19 pandemic: an international perspective. Br J Ophthalmol. 2020;104(11):1477-1481.
4. Armitage WJ, Tullo AB, Larkin DFP. The first successful full-thickness corneal transplant: a commentary on Eduard Zirm’s landmark paper of 1906. Br J Ophthalmol. 2006;90(10):1222-1223.
5. Gain P, Jullienne R, He Z, et al. Global survey of corneal transplantation and eye banking. JAMA Ophthalmol. 2016;134(2):167-173.
6. Ang M, Wilkins MR, Mehta JS, Tan D. Descemet membrane endothelial keratoplasty. Br J Ophthalmol. 2016;100(1):15-21.
7. Yu AC, Spena R, Pellegrini M, Bovone C, Busin M. Deep anterior lamellar keratoplasty: current status and future directions. Cornea. 2022;41(5):539-544.
8. Colby K. Descemet stripping only for Fuchs endothelial corneal dystrophy: Will it become the gold standard? Cornea. 2022;41(3):269-271.
9. Trefoil Therapeutics announces positive phase 2 study data showing corneal regeneration and vision recovery following Descemet stripping only (DSO) surgery. September 29, 2022. Accessed September 8, 2023. https://eyewire.news/news/trefoil-therapeutics-announces-positive-phase-2-study-data-showing-corneal-regeneration-and-vision-recovery-following-descemet-stripping-only-dso-surgery?c4src=article:infinite-scroll
10. Kinoshita S, Koizumi N, Ueno M, et al. Injection of cultured cells with a ROCK inhibitor for bullous keratopathy. N Engl J Med. 2018;378(11):995-1003.
11. Numa K, Imai K, Ueno M, et al. Five-year follow-up of first 11 patients undergoing injection of cultured corneal endothelial cells for corneal endothelial failure. Ophthalmology. 2021;128(4):504-514.
12. Ong HS, Ang M, Mehta JS. Evolution of therapies for the corneal endothelium: Past, present and future approaches. Br J Ophthalmol. 2021;105(4):454-467.
13. Mehta JS, Kocaba V, Soh YQ. The future of keratoplasty: Cell-based therapy, regenerative medicine, bioengineering keratoplasty, gene therapy. Curr Opin Ophthalmol. 2019;30(4):286-291.
14. Ljubimov AV, Saghizadeh M. Progress in corneal wound healing. Prog Retin Eye Res. 2015;49:17-45.
15. Deng SX, Dos Santos A, Gee S. Therapeutic potential of extracellular vesicles for the treatment of corneal injuries and scars. Transl Vis Sci Technol. 2020;9(12):1.
16. Jacob S, Patel SR, Agarwal A, Ramalingam A, Saijimol AI, Raj JM. Corneal allogenic intrastromal ring segments (CAIRS) combined with corneal cross-linking for keratoconus. J Refract Surg. 2018;34(5):296-303.
17. Kalas T, Gunn D. Corneal allogenic intrastromal ring segment implantation for the treatment of post-LASIK keratectasia. Presented at: Royal Australian and New Zealand College of Ophthalmologists Congress; February 26-March 1, 2022; Brisbane, Australia.
18. Keranatural. VisionGift. Accessed August 10, 2023. https://www.visiongift.org/transplant/keranatural
19. Auffarth GU, Son HS, Koch M, et al. Implantation of an artificial endothelial layer for treatment of chronic corneal edema. Cornea. 2021;40(12):1633-1638.
20. Abusayf MM, Tan GS, Mehta JS. Pull-through insertion of EndoArt for complex eyes. Am J Ophthalmol Case Rep. 2023;32:101878
21. Litvin G, Klein I, Litvin Y, Klaiman G, Nyska A. CorNeat KPro: ocular implantation study in rabbits. Cornea. 2021;40(9):1165-1174.
22. Rafat M, Jabbarvand M, Sharma N, et al. Bioengineered corneal tissue for minimally invasive vision restoration in advanced keratoconus in two clinical cohorts. Nat Biotechnol. 2023;41(1):70-81.